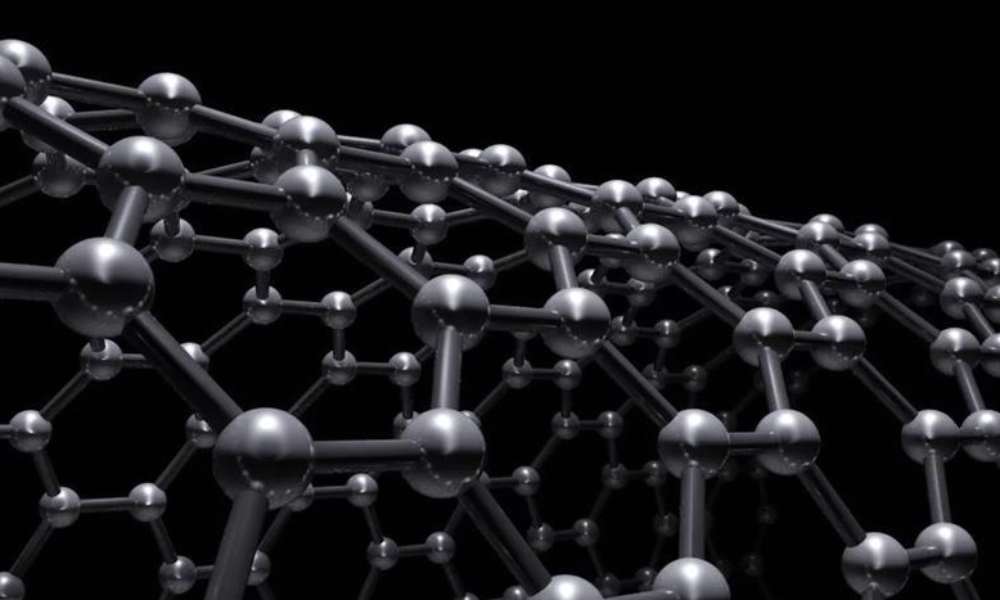
Carbon: From Soft to Superhard
The Main Carbon Allotropes and Their Properties
A. J. Li
CARBON IS PERHAPS THE MOST IMPORTANT ELEMENT OF ALL. Heralded as essential to all life due to its ability to bond in many different ways, there is a whole branch of chemistry dedicated to the study of carbon chemistry – organic chemistry. However, the purpose of this article is not to explore how carbon bonds to other elements, but how it can bond with itself through allotropy. Allotropes are variations of the same pure chemical element, differing in their structure and bonding. Due to these differences, allotropes of the same element often have very different physical and chemical properties. Some of the most well-known allotropes of carbon are diamond and graphite.
Diamonds:
A symbol of wealth, love, and luxury, diamonds are the hardest naturally occurring substance (on Earth, at least, but that’s a story for another time). The structure of diamonds fully takes advantage of carbon’s ability to bond to four other atoms, resulting in a crystal lattice of carbons single-bonded to three other carbon atoms.
Diamonds only form under the most extreme conditions to create unique bonding arrangements. They are formed naturally over millions of years 150-200 kilometres underground, under 50,000 times the pressure at the surface of the Earth, and at a temperature of about 1600°C. These extreme conditions happen to match a planet 40 light years away named 55 Cancri e, where one-third of the planet’s mass could potentially be made up of diamonds. Diamonds are commonly used for jewellery and drill bits in mining due to their extreme strength.
Graphite:
Graphite differs from the structure of diamonds in that each carbon is covalently bonded to only three adjacent carbon atoms. This leaves one electron free to move around, which turns into a sea of delocalised electrons when looking at an extended structure of graphite.
The delocalised electrons in graphite make it an excellent conductor of electricity, the opposite of diamond which is an insulator since there are no free electrons. Perhaps the most famous use of graphite is in pencil lead, which also demonstrates another one of its unique properties. As seen from the diagram above, graphite seems to exist in 2D planes, stacked on top of each other. This is due to the carbon atoms bonding only to three other atoms instead of four, and so it is more favourable for the bonds to form in a 2D flat plane. When a pencil is used, the pressure from writing is enough to strip layers off of the graphite lead in the pencil to leave marks on the paper. The same idea leads to graphite being used as a dry lubricant, which is convenient when viscous lubricants like oil and grease cannot be used. The individual layers can easily slide over each other to create a lubricating effect, even being used by string musicians when changing instrument strings. Graphite, through the use of a pencil, is applied to the wooden bridge of the instrument, which would not have been possible with oil or grease. Another application of graphite is in the core of nuclear reactors. Due to the strength of the covalent bonds, graphite has extremely high melting points of above 3600°C. Its ability as a neutron absorber thus makes it suitable in the core of nuclear reactors to stop or slow nuclear reactions that take place due to neutron chain reactions.
Graphene:
Graphene is a single layer from the graphite structure, as close as we can get to 1D.
Retaining many of the properties of graphite, especially its excellent conductivity, graphene has many applications in microtechnological areas such as transparent solar cells, and smart tech such as smart windows, which can control both heat and light transmission, as well as electronic displays. Graphene is also the precursor to the following more exotic allotropes of carbon, which open the door to nanotechnology.
Carbon Nanotubes:
The study of carbon nanotubes is a relatively new area of study, uniting physics and chemistry disciplines in a fast-moving research environment. Essentially, carbon nanotubes are formed from rolling graphene into a tube structure.
This creates a material with remarkable properties: excellent heat and electrical conductivity, among the highest tensile strengths in existing materials, and chemical inertness. Due to their extremely small size and weight, they have wide-ranging applications and enormous potential to revolutionise many industries from all levels of technology to biomedical applications. They can also be spun into fibres to form woven fabrics and textiles, creating extremely efficient transmission cables, and even body and vehicle armour. However, one of its main strengths is also the main issue inhibiting further research on this exciting material: its small size. At such small sizes, it becomes hard to produce in high purities as even the smallest disturbance or contaminant in the creation process can massively impact the final product.
Buckminsterfullerene:
Perhaps the most exotic allotrope of carbon, buckminsterfullerenes have a unique shape resembling that of a football.
Due to this resemblance, they are also known as “BuckyBalls.” The bonding in buckminsterfullerene creates the fused ring structure where each carbon is bonded to three adjacent carbons. However, unlike graphene, the bonding forms a 3D ball shape, with each unit needing 60 carbons to complete the football shape. Buckminsterfullerene is also the only allotrope on this list to exist as individual units (C60), whereas the other allotropes exist in crystalline structures. This leads to the property of buckminsterfullerene being soluble in organic solvents. One of the main applications of buckminsterfullerene is in the medical field. Due to the space inside the “football” structure, atoms and small molecules can be trapped inside, allowing for advanced delivery of medicines and genes to different parts of the body through this carrier. Buckminsterfullerene is also reactive to light and has applications in treating cancer. The buckyballs make their way to cancer cells, where they then react with light radiation to convert molecular oxygen into a more reactive species, which then targets and kills cancer cells. This approach reduces damage to surrounding healthy tissue during treatment compared to more conventional methods using chemotherapy for example.
This is by no means a definitive list of carbon allotropes, there exist many more allotropes with their own unique chemical and physical properties. The plethora of applications and unbridled potential seen with just the four allotropes explored in this article reveal the amazing properties of carbon in an interdisciplinary scientific research area. With every year that passes, carbon nanotubes and other exotic allotropes are closer to enhancing our everyday lives and making the world a better place.